-
iestinstrument
Synergistic Surface Reconstruction Of Ultrahigh Nickel Cathode Materials By Ultrafast Joule Heating Method In Seconds
Corresponding author: Dong Jinyang, Su Yuefeng, Chen Lai
Corresponding unit: Beijing Institute of Technology, Innovation Center of Beijing Institute of Technology
Instruments used: IEST PRCD3100 Powder Resistivity & Compaction Density Tester, SPFT-2000 Single Particle Mechanical Properties Test System
1. Research background
Lithium-ion batteries (LIBs) play a vital role in the field of modern energy storage and have a profound impact on the development of portable electronic devices, electric vehicles and renewable energy technologies. These batteries are recognized for their high efficiency, high energy density and rechargeability. The cathode material is a key factor in determining the energy density and cost-effectiveness of LIBs, and a variety of cathode materials including layered, spinel and olivine structures are being studied. Layered ternary cathode materials have significantly improved the performance of next-generation batteries due to their low cost, high energy density and fast charging capability. However, despite the progress, high nickel cathode materials with a capacity close to mAh g-1 have not met industrial needs, highlighting the need for further innovation in cathode material development. In high nickel cathode materials, high nickel concentration significantly exacerbates volume and surface degradation, and the significant expansion and contraction of the lattice induces mechanical stress and strain during the cycle, resulting in lattice distortion and microstructural changes, which in turn produce microcracks and compromise the integrity of the cathode. In addition, the high reactivity of Ni4+ ions leads to unfavorable interactions with the electrolyte, consuming Li+ ions while increasing the cathode/electrolyte interface (CEI) layer of interfacial impedance, ultimately leading to irreversible capacity loss. These degradation mechanisms associated with ultra-high nickel lithium-ion batteries lead to significant reductions in their cycle life and safety, especially at high temperatures and high charge cut-off voltages.
2. Work Introduction
Recently, the team of Chen Lai, Dong Jinyang, and Su Yuefeng from Beijing Institute of Technology quickly promoted synergistic surface reconstruction in ultra-high nickel cathode materials through ultra-fast Joule heating, solving the major challenges of structural and interface degradation during the cycle. Density functional theory (DFT) calculations, synchrotron X-ray absorption spectroscopy (XAS), and single-particle crush tests confirmed the establishment of a stable crystal framework and lattice oxygen, which mitigated the H2-H3 phase transition and improved structural reversibility. In addition, the Sc mixing process showed a pinning effect on the grain boundaries, enhanced the Li+ diffusion kinetics, and reduced the mechanical strain during cycling. The cation mixed phase formed in-situ at the grain boundaries also formed a strong cathode/electrolyte interface, effectively reducing interfacial side reactions and transition metal dissolution. These synergistic improvements reduce particle cracking and surface/interface degradation, thereby enhancing rate performance, structural integrity, and thermal stability. As a result, the optimized Sc-modified ultra-high nickel cathode (Sc-1) showed a capacity retention of 93.99% after 100 cycles at 1C (25°C) and a capacity retention of 87.06% after 100 cycles at 1C (50°C), indicating excellent cycling and thermal stability. This study presents a one-step, multi-purpose modification approach to extensively analyze the microstructural and interfacial properties of high-nickel layered cathode materials (NCMs), and these results emphasize the potential of ultra-high nickel cathodes as viable candidates for next-generation advanced lithium-ion batteries (LIBs) for electric vehicles (EVs). The related work is titled “Synergistic surface restructuring and cation mixing via ultra-fast Joule heating enhancing ultra-high nickel cathodes for advanced lithium-ion batteries” in the Journal of Energy Chemistry.
3. Presentation of content
Figure 1. Schematic representation of heterostructured anode materials prepared by the Joule heating synthesis method
Figure 1 shows a schematic diagram of the heterostructured cathode materials prepared by the Joule heating synthesis method, as well as XRD refinement maps, powder resistivity, scanning electron microscopy (SEM) images, and Ni 2p X-ray photoelectron spectroscopy (XPS) spectra of the undoped Sc (Sc-0) and Sc-doped (Sc-1) samples. These images and data reveal the effect of Sc mixing on the crystal structure, resistivity, and surface chemical state of the samples.The XRD refinement results show that the Sc-doped samples display stronger Li+/Ni2+ cation mixing, which is attributed to the structural modification of Sc in the lattice of the high-Ni cathode material, especially in the surface and near-surface regions. In addition, combined with PRCD3100 detection showed a decrease in the powder resistivity of the Sc-1 samples, suggesting that Sc surface mixing may improve the multiplicity performance.SEM images showed that all the samples consisted of spherical secondary particles of approximately 10 μm in size, suggesting that the surface mixing of Sc has little effect on the particle morphology. XPS analyses confirmed the form of Sc present in the Sc-1 samples, and showed a significant increase in the Ni2+and Ni3+ coexistence, suggesting that Sc mixing contributes to the successful mixing of Ni sites during Joule heating, leading to the formation of a cationic mixed layer, which reduces the oxidation state of Ni and lengthens the Ni-O bond.
Figure 2. Local structural changes in pristine Sc-0 and modified Sc-1 samples
Figure 2 Local structural changes of the pristine Sc-0 and modified Sc-1 samples were directly observed by high-resolution transmission electron microscopy (HRTEM), fast Fourier transform (FFT) and inverse fast Fourier transform (iFFT) images. The images show that the Sc-0 sample exhibits a pure laminar structure from the surface to the bulk phase, whereas the lattice fringes of the Sc-1 sample are divided into two distinct regions: a thin mixed phase (NiO-like rock salt phase and a laminar phase) on the surface (region b-1) and a well-structured laminar structure in the bulk phase (region b-2). These findings are consistent with the XRD and XPS analyses, indicating that the reconstruction of the surface mixed phase requires a specific level of Sc mixing to achieve the appropriate thickness.EDS mapping of Sc-1 further confirms the successful mixing of Sc into the lattice. The Sc mixing-induced surface reconstruction layer produces a pegging effect that mitigates the significant contraction of the c-axis during the deep delithiation process, effectively reducing the transition from the R-3m phase to the structural degradation of the disordered rock salt phase Fm3m. Thus, the cationic hybrid layer generated by Sc surface mixing acts as a protective barrier for the material during cycling, enhancing its durability and electrochemical performance.
Figure 3. Initial chargedischarge curves, multiplicity performance, dQ/dV curves, and cycling performance of Sc-0 and Sc-1 samples at 25°C and 50°C
Figure 3 demonstrates the initial charge/discharge curves, multiplicity performance, dQ/dV curves, and cycling performance of Sc-0 and Sc-1 samples at 25°C and 50°C. At 25°C, the Sc-1 sample exhibits a higher initial charge/discharge capacity and a slightly lower initial coulombic efficiency than Sc-0, which may be attributed to the small amount of volatilization and loss of surface-active lithium due to Joule heating during the Sc modification process. The multiplicity performance test showed that Sc-1 displayed higher specific capacity than Sc-0 at different current densities, indicating that Sc mixing contributes to the improvement of the multiplicity performance. dQ/dV curves showed a decrease in the polarization of Sc-1 with the increase of multiplicity, indicating its better performance at higher multiplicity. The cycling performance test results show that the capacity retention of Sc-1 after 100 weeks of cycling is significantly higher than that of Sc-0, indicating better cycling stability. These results indicate that Sc mixing and surface reconstructing significantly improve the electrochemical performance of ultra-high nickel cathode materials, especially at high temperatures.
Figure 4. Single-particle crush tests, cyclic voltammetry (CV) curves and in situ electrochemical impedance spectroscopy (EIS) results
Figure 4 evaluates the mechanical and electrochemical properties of Sc-0 and Sc-1 samples through single-particle crush tests, cyclic voltammetry (CV) curves, and in situ electrochemical impedance spectroscopy (EIS) results. The single particle crush test showed that Sc-1 required more pressure before the crushing point, indicating a higher compressive strength, which contributes to the overall electrochemical performance of the cell. CV tests with different sweep speeds showed that Sc-1 has a larger Li+ diffusion coefficient associated with enhanced Li+ mobility, which contributes to improved cycling stability at high current densities. In-situ EIS measurements further showed that Sc-1 has a lower interfacial impedance and a more stable charge transfer impedance (Rct) than Sc-0, indicating better interfacial stability and enhanced charge transfer kinetics.
Figure 5. In-situ XRD pattern
Figure 5 Structural evolution of Sc-0 and Sc-1 electrodes during the initial charging process as shown by the in situ XRD patterns and the corresponding stacked plots of (003) and (101) peaks. (The shift of the (003) peak indicates the change of the c-axis lattice parameter, while the shift of the (101) peak reflects the change of the a-axis lattice parameter.The (003) peak in the Sc-1 sample is shifted by a maximum of 0.82° during charging and is accompanied by a slow phase transition from the H1 and H2 to H3 phases. This contrasts with the abrupt shift of the (003) peak in Sc-0, which reaches a maximum shift of 1.02°, suggesting that the lattice distortion is more pronounced in the absence of scandium mixing. The smaller peak displacement in Sc-1 suggests that the surface Sc mixing effectively modulates the phase transition, thereby enhancing the structural stability.
Figure 6. Profile SEM images, HRTEM and corresponding FFT/iFFT images of Sc-0 and Sc-1 samples after 100 weeks of cycling.
Figure 6 shows the profile SEM images, HRTEM and corresponding FFT/iFFT images, as well as time-of-flight secondary ion mass spectrometry (TOF-SIMS) images and Ni L-edge soft X-ray absorption spectroscopy (XAS) spectra of the Sc-0 and Sc-1 samples after 100 weeks of cycling. These images and spectra confirmed the enhanced chemical and mechanical stability of the Sc-1 anode after cycling.The Sc-0 samples showed pulverization and accumulation of side-reaction products after cycling, whereas Sc-1 maintained the structural integrity and reduced the side-reactions.The sectional SEM images of the Sc-1 samples showed that microcracks were effectively suppressed in their formation and extension, which was attributed to the surface reconstruction induced by Sc mixing, the mitigated the lattice shrinkage and expansion during the cycling process.TOF-SIMS results showed that the CEI layer of Sc-1 was thinner, suggesting that there were fewer side reactions between the Sc-1 anode and the electrolyte. Soft XAS spectra showed that the oxidation state of Ni was more stable in the cycled Sc-1 samples, suggesting that Sc-1 maintained the lattice structure more effectively after cycling.
Figure 7. Effect of Sc mixing on the crystal structure and electronic structure of ultra-high nickel cathode materials
Figure 7 demonstrates the effect of Sc mixing on the crystal structure and electronic structure of ultra-high nickel cathode materials through theoretical calculations and simulation results. Density Functional Theory (DFT) calculations evaluated the formation energy of Sc mixing, and the results showed that Sc mixing at the Ni sites has a lower formation energy, suggesting that Sc mixing into the transition metal layer is the most energy-efficient structure. In addition, Sc mixing significantly improves the stability of the lattice oxygen environment and reduces the formation of oxygen vacancies. Density of electronic states (DOS) analysis shows that Sc mixing introduces new electronic states and increases the electron density near the Fermi energy level, which contributes to the reduction of the band gap and enhances the electronic conductivity. Differential charge density analysis shows that the Sc-O bond is more stable than the Ni-O bond, which is important for improving the structural stability of the anode.
Figure 8. Effect of Sc mixing on lithium ion concentration distribution and release
Figure 8 demonstrates the effect of Sc mixing on Li-ion concentration distribution and stress through COMSOL simulations. In the initial charge/discharge cycling of the unmodified Sc-0 samples, the reduction of the Li-ion diffusion coefficient leads to a significant concentration difference, with the Li-ion concentration in the bulk phase being higher than that at the surface. This difference leads to a severe stress gradient during prolonged cycling at high cutoff voltages. In contrast, the Sc-modified samples showed improved diffusivity, resulting in a more uniform distribution of lithium concentration and a reduced stress gradient. The Sc-modified samples maintained a stable ion distribution even after prolonged cycling. This enhanced performance is attributed to the synergistic effect of surface reconstruction and cation mixing, which increases the lithium diffusion coefficient, thereby supporting efficient ion transport and long-term cycling stability of the material.
4. Summary and outlook
In conclusion, this study demonstrates synergistic surface reconstruction of ultra-high nickel cathode materials by ultrafast joule heating method in seconds. This approach not only accelerates the synthesis of the cathode material, but also permits the control of key structural features that enhance its electrochemical performance. During the Sc surface mixing process, an electrochemically inert cationic hybrid layer was strategically introduced, which was integrated into the lamellar structure. The non-equilibrium environment created by the ultra-fast Joule heating process is essential to facilitate this surface reconstructing, which significantly improves the structural/interfacial stability and mechanical integrity of the UHN layered cathode. Sc mixing shows a “pinning effect” on the grain boundaries of the cathode particles, which is essential to improve the Li+ diffusion kinetics by reducing the mechanical strain experienced by the cathode during the cycling process. Li+diffusion kinetics by reducing the mechanical strain experienced by the anode during cycling. By promoting faster Li+ migration, the material is able to withstand higher charge/discharge rates, thus enhancing overall performance. In addition, the cationic mixed layer formed in-situ at grain boundaries establishes a robust anode/electrolyte interface, which is critical for interfacial reactions. Specifically, this protective layer inhibits side reactions between the anode and electrolyte and prevents the dissolution of transition metals (TMs) during cycling. Advanced characterization techniques including STEM and TOF-SIMS confirmed the reduction of TM dissolution in the modified anode. Consequently, these synergistic effects allowed Sc mixing to mitigate particle cracking and minimize surface and interface degradation, resulting in enhanced rate performance, structural stability, and thermal stability. In conclusion, this study introduces an innovative and efficient design and synthesis strategy for the fabrication of low-cost, high-performance anode materials. The combined benefits of such materials are particularly important for applications requiring high energy density and operational stability, such as electric vehicle batteries.
5. References
Haoyu Wang, Jinyang Dong, Meng Wang, Yun Lu, Hongyun Zhang, Jinzhong Liu, Yun Liu, Na Liu, Ning Li, Qing Huang, Feng Wu, Yuefeng Su, Lai Chen. Synergistic surface restructuring and cation mixing via ultrafast Joule heating enhancing ultrahigh-nickel cathodes for advanced lithium-ion batteries, Journal of Energy Chemistry, 2024, ISSN 2095-4956.
6. Related Testing Equipment Recommendation
IEST Powder Resistivity & Compaction Density Measurement System(PRCD3100)
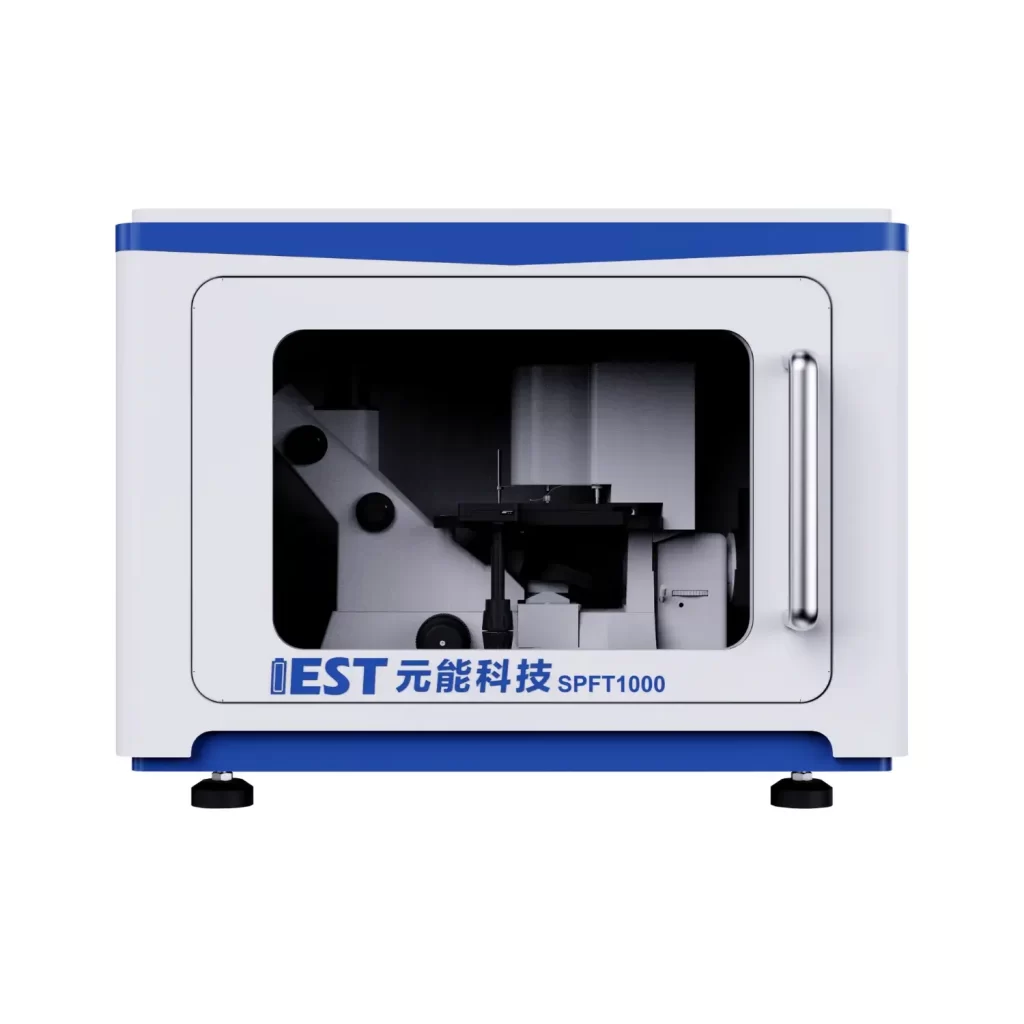
Application:
- Testing the crushing strength of battery material particles
- Can be used to evaluate the pressure resistance of the material
- Guide the rolling process
- Materials with high mechanical strength will have better subsequent cycle stability
Subscribe Us
Contact Us
If you are interested in our products and want to know more details, please leave a message here, we will reply you as soon as we can.