1. Introduction
With the rapid development of the lithium-ion battery industry, concerns over safety and stability during battery use have grown significantly. The primary causes of performance degradation in lithium-ion batteries can be summarized as loss of active lithium, loss of active materials, and polarization losses. In practical battery development, from raw materials through electrode fabrication to final cell assembly, the characteristics at each level and their evolution will affect battery performance. Therefore, establishing multi-level correlations from powder materials to electrode sheets to complete cells is crucial in lithium-ion research and is currently a key focus for battery scientists. This article shares exploratory insights into correlating powder resistivity with electrode resistivity, aiming to identify root causes early in the manufacturing chain and reduce time/cost waste.
As a self-developed instrument company, IEST Instrument not only focuses on the performance of its machines but also strives for breakthroughs in mechanistic analysis methods. In our previous work, we designed an experiment using five powders with different resistivities, fixing the solid content at 53.3%. We prepared slurries at a mass ratio of 96.5:1.5:2 (active material: conductive carbon: PVDF), then carried out systematic coating, calendaring, punching, and cell assembly. We analyzed conductivity changes at each level—powder, slurry, electrode sheet, and full cell—to explore their interrelations. Figure 1 illustrates these multi-level correlations. The results showed that, from powder to slurry, the introduction of additives, solvents, and process variability prevented a perfectly consistent conductivity trend. At the cell level, DC internal resistance is influenced by electronic resistance, charge-transfer resistance, and lithium-ion diffusion resistance among components, leading to further inconsistencies. However, materials with large powder-level resistivity differences (samples 1 and 5) still showed consistent trends across levels.
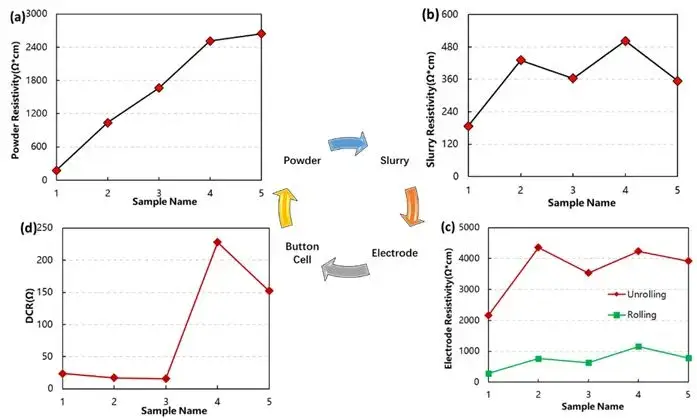
Figure 1. Multi-level correlation analysis of lithium battery.
The microstructure and morphology at each stage—from raw powder, through premixed powder and slurry, to coated electrode—differ significantly, as shown in Figure 2. For powder-level conductivity, which includes only active particles, the conductivity under a given compaction can be expressed as σAM =σ0*φ/τ
where σ0* is the intrinsic conductivity of the powder, φ is the particle volume fraction under compaction, and τ is the tortuosity of particle connectivity, related to contact conditions between particles. Better connectivity and larger contact areas yield smaller τ . During powder resistivity tests, increasing pressure raises compaction density, increases φ, improves connectivity, reduces τ, and thus increases conductivity.
From the pre-mixed powder state to the slurry and then to the electrode, conductive agents and binders are introduced into the active particle powder. Conductive agents facilitate the construction of electron transport networks, while binders increase electron transport impedance. The conductivity of mixed powders, slurries, or electrode coatings can be divided into two components:
- The conductive network formed by interconnected active particles.
- The conductive network formed by the conductive agents.
Additionally, interfacial contacts between these two networks also contribute to electron transport.
The effective conductivity can thus be expressed as:
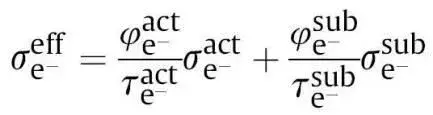
Among them, σeffe is the effective conductivity; φact is the volume fraction of active particle powder; τact is the connectivity tortuosity of active particle powder. The better the connectivity and the larger the contact area, the smaller the tortuosity τ of the electron conduction path; σact is the electronic conductivity of the active particle itself. φsub is the volume fraction of conductive agent powder, τsub is the connectivity tortuosity of conductive agent powder, and σsub is the electronic conductivity of the conductive agent particle itself. Generally, the conductivity of active particles itself is much lower than that of conductive agents, especially positive electrodes. Therefore, the electronic conductivity contributed by active particles is very small, and conductive agents are the main path for electron conduction. Therefore, the distribution state and connectivity of conductive agents are the main factors affecting electronic conductivity. Compared with pure powder, the introduction of conductive agents and binders into the slurry directly affects the evaluation results of electronic conductivity. From the mixed powder premix to the slurry and then to the electrode, the correlation of electronic conductivity is mainly related to the distribution state and connectivity of the components, especially the connected network of the conductive agent. If this conductive network can be preserved during the process, the conductivity of each level will be completely correlated.
2. Correlation Between Pre-Mixed Powder and Electrode Sheet Resistivity
In the exploration of correlations across different hierarchical levels (Figure 1), it was observed that the relationship between the powder-level and electrode sheet-level resistivity exhibited inconsistencies (significantly influenced by conductive agents), whereas the correlation from slurry to electrode sheets remained fully consistent. This discrepancy primarily arises from the introduction of conductive agents and binders during the transition from powder to slurry to electrode sheets, which directly impacts the evaluation of electronic conductivity. To clarify these effects, laboratory experiments were designed using NCM materials as the primary component, referencing the pre-mixing stage of dry mixing processes. Two formulations were prepared:
- NCM:PVDF = 19:1
- NCM:PVDF:SP = 18:1:1
These mixed powders were thoroughly homogenized, and resistivity assessments were conducted using IEST PRCD Series to quantify conductivity differences before and after mixing. As shown in Table 1:
-
Blending PVDF (a poorly conductive binder) with NCM resulted in deteriorated conductivity compared to pure NCM powder.
-
Adding SP (a highly conductive agent) significantly improved conductivity, confirming that enhanced conductivity in cathode electrode sheets is primarily attributable to conductive agents.
To probe the correlation between powder resistivity and electrode resistivity, a promising approach involves analyzing the resistivity of pre-mixed powders. IEST Instrument is currently refining its proprietary coin cell assembly line and plans to integrate in-house mixing, coating, and calendering processes to validate the feasibility of this methodology.
Table 1. Resistivity changes in pre-mixed powders
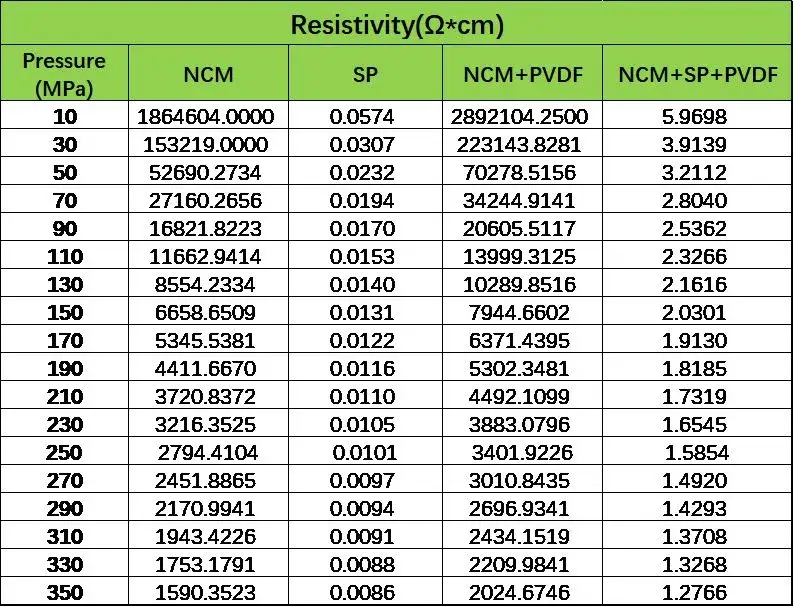
3. Exploration of the Correlation between Electrode Resistivity and the Resistivity of the Coating‐Layer Powder
In premixed‐powder experiments, one may notice that the way conductive additive and binder are introduced at the electrode level differs markedly from the pure‐powder premix. After slurry‐level mixing, PVDF exists in a sol state and a solvent is introduced; consequently, once the electrode sheet is dried, its state differs clearly from that of the premixed powder. Colleagues at IEST Instrument raised questions about this discrepancy, so we designed an electrode sheet level scrape test: we scraped off the coating from the electrode sheet, crushed the scraped powder, and measured its resistivity, then used BER and PRCD series instruments to evaluate its correlation with the electrode resistivity.
In this part of the experiment, two uncalendered electrode sheets, NCM and LCO, were selected as samples, and the resistivity of different sites of the samples was tested under a pressure of 25MPa to clarify that the coating uniformity of the selected electrode sheet is reasonable and avoid introducing too large differences in each site during the voltage transformation test stage. Figure 3 shows the schematic of the BER series instrument alongside the single‐point test results for the selected films. Six points at distinct positions on each film were tested, and the coefficient of variation (COV) of their resistivities was calculated. All electrode sheets exhibited a COV below 5%, preliminarily confirming their good uniformity and deeming them suitable as sample films for this experiment.
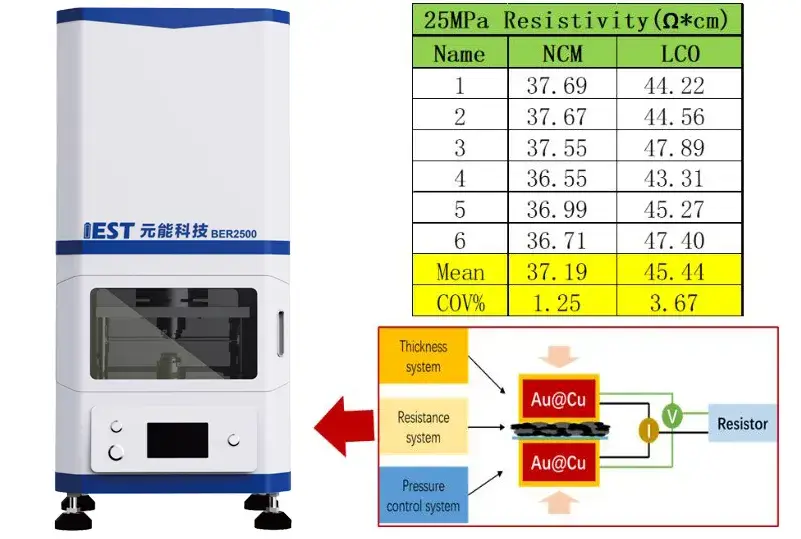
Figure 3. BER series equipment schematic and electrode sheet single point measurement results
According to the experimental design concept, both types of electrode sheets were subjected to a powder scraping procedure: the coating layer was scraped from the surface of the current collector, then the collected powder was milled to ensure sampling consistency during resistivity measurement. Figure 4 shows the schematic of the PRCD series instrument and the measured powder resistivity of the coating‐layer. From the curves, the coating‐layer powder displays the same trend as the pure powder: resistivity decreases progressively as applied pressure increases.
To further clarify any differences, we compared the electrode resistivity with the coating‐layer powder resistivity; Table 2 summarizes the measured results. The data reveal no order of magnitude difference between the two, and in both states LCO exhibits higher resistivity than NCM. suggesting that our approach is reasonable and effective.
However, given the limited sample selection, the current validation is not yet comprehensive. We must design additional experiments: using the same active material under different process conditions to verify these findings. We are also considering, in addition to scraping the coating layer, directly simulating the coated slurry (either dried or simply oven‐dried) and then milling it, to correlate with electrode sheet performance. If this proves feasible, it may be possible to predict electrode level performance without actually coating the foil. IEST Instrument next internal step will be to design experiments to explore each of these possibilities in turn.
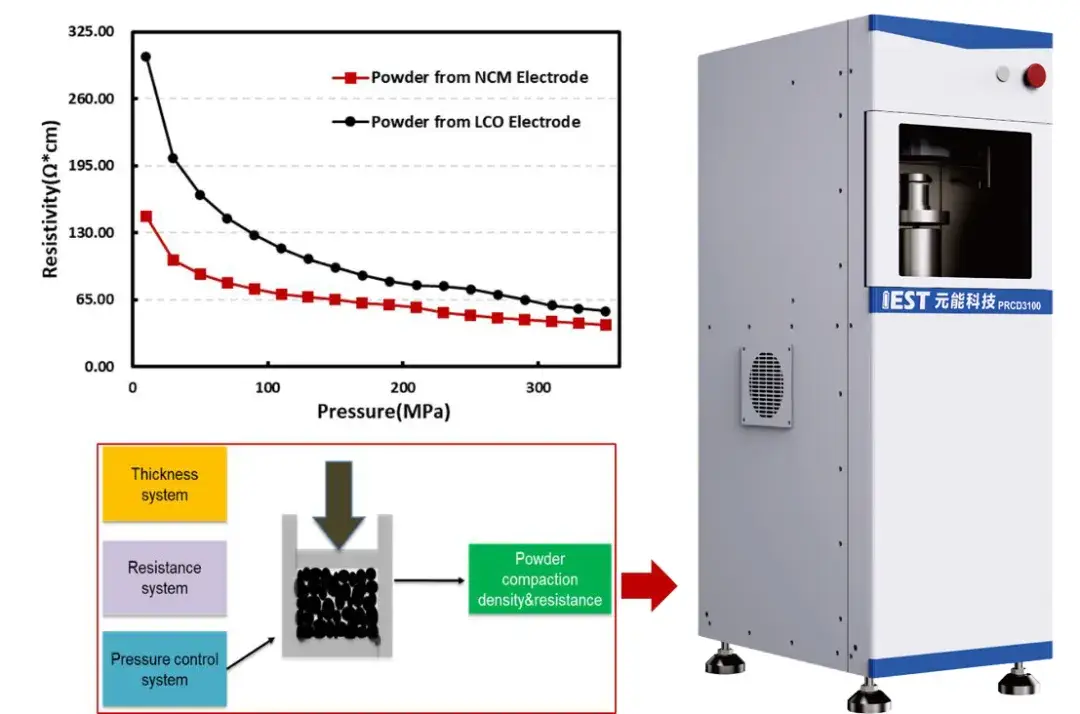
Figure 4. Schematic diagram of the PRCD series instrument and the results of the powder resistivity of the electrode coating layer
Table 2. Comparison of electrode resistivity and coating-layer powder resistivity
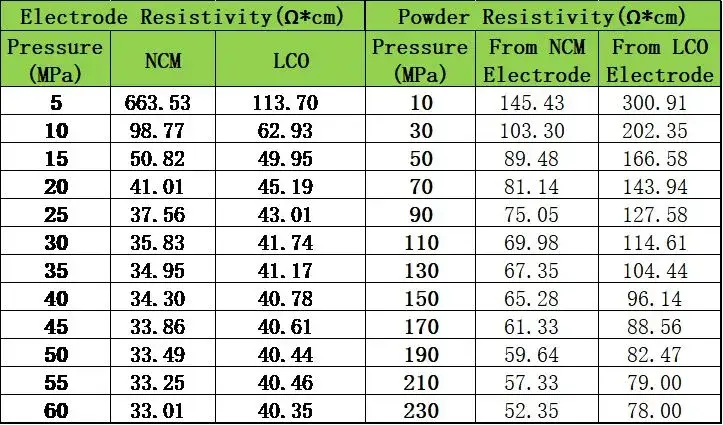
4. Summary
With the rapid pace of industry development, the correlations between different levels of lithium-ion batteries have become a key focus. In particular, the electrical conductivity of materials is closely linked to cell performance and safety assessments. Drawing on our laboratory’s testing experience, this paper offers new approaches to exploring the relationship between powder resistivity and electrode-film resistivity. Through premixing and evaluation at the powder stage, we clarified the direct impact of introducing binder and conductive additive on resistivity. Furthermore, by considering the effects of solvent addition and differences in binder state, we performed comparative tests on coating-layer powder scraped from finished electrode films. Our preliminary evaluation indicates that this method can serve as an effective means of probing inter-level correlations. In the next phase, YuanNeng will refine the experimental plan to facilitate a more systematic correlation assessment.
5. References
[1] Yang Shaobin, Liang Zheng. Principles and Applications of Lithium-Ion Battery Manufacturing Processes.
[2] MikoWoo@Ideallife. Fundamentals of Lithium-Ion Battery Electrode Theory and Processing.